Research Synopsis (with Animations!)
[Animations provided courtesy of Robert Hurt and Tim Pyle, SSC.]
Q: What are all these papers?
Using advanced techniques with new space-based telescopes that detect light at infrared wavelengths, my collaborators and I are providing answers to many questions about the early development of stars and planets. (Higdon et al. 2004)
Spitzer Launch
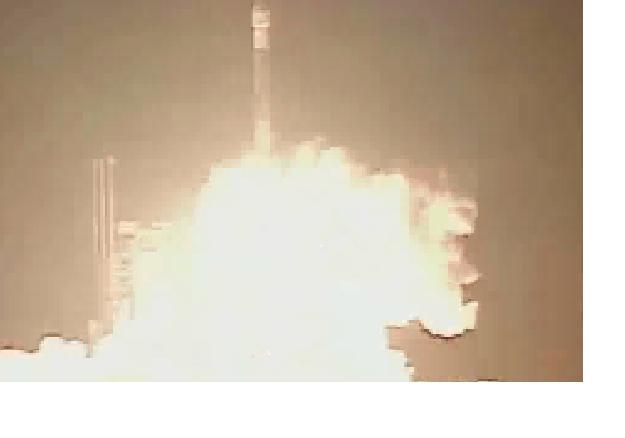
The Orbit of the Spitzer Space Telescope
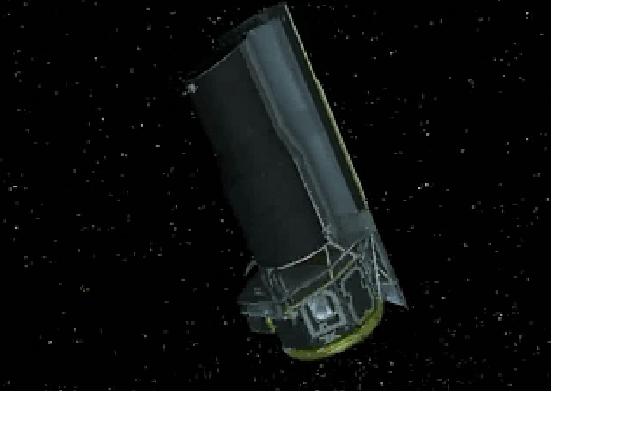
Q: Why use an infrared space telescope?
The animation below shows the stellar nursery IC 1396. It begins in optical wavelengths in the range of Hubble. Note that the cloud is nothing but a veil of darkness blotting out the background stars. As we move into the infrared, we see the star formation region come to life.
Lifting the Veil with Spitzer
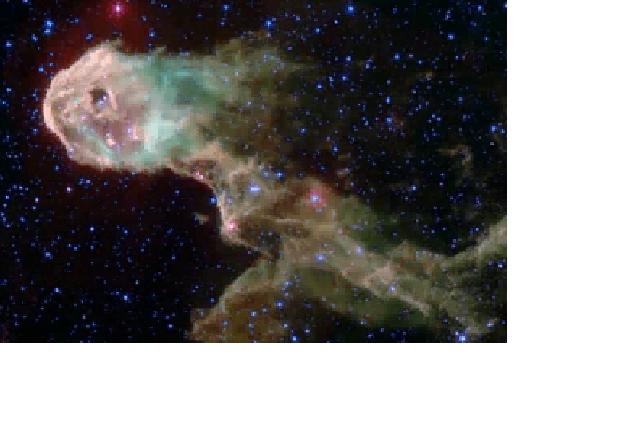
Q: What's the general process for creating stars and planets?
The (relatively) short answer:
Imagine that in a small section of the galaxy lies a cloud of gas, made up of molecular hydrogen with some mix of other light molecules (Neufeld et al. 2006a), (Neufeld et al. 2006b). If some small part of that cloud is compressed, it may start to collapse on itself. As this ball of gas collapses to form a protostar, surrounded by an envelope of colder gas, the surrounding material is also mixed and pulled in (Watson et al. 2004). If this material has any initial spin, it will rotate even more quickly as it falls, conserving angular momentum. While the star is still growing to its final size, much of the surrounding material is coalescing into a relatively flat disk that is spinning about the central protostar, perhaps firing off bipolar jets into the ambient medium. Ice crystals bring in water and other molecules from the envelope to the disk in a violent cascading waterfall that vaporizes much of the incoming material and distributes it around the new circumstellar disk (Watson et al. 2007b), (Zasowski et al. 2008),
(van Kempen et al. 2010), (Green et al. 2013b).
Accretion and Jets
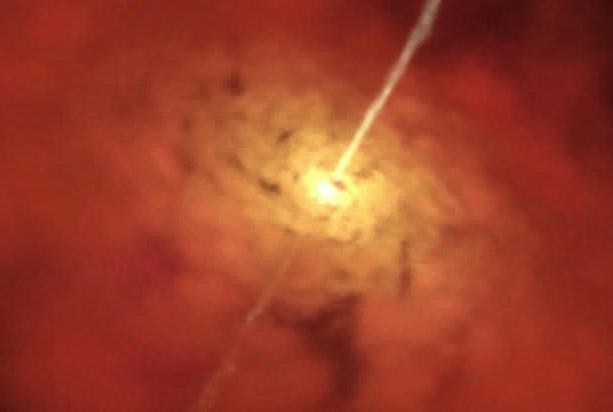
Finally, the envelope has been more or less cleared from around the star and a spinning disk has formed. The disk grains collide and stick together in spots to form larger grains and crystalline structures, slowly building into planetessimals (Furlan et al. 2005b), (Sargent et al. 2006), (Watson et al. 2007a), (Sargent et al. 2008a), (Sargent et al. 2008b), (Furlan et al. 2008), (Sturm et al. 2010).
Ice Sticks to Grains
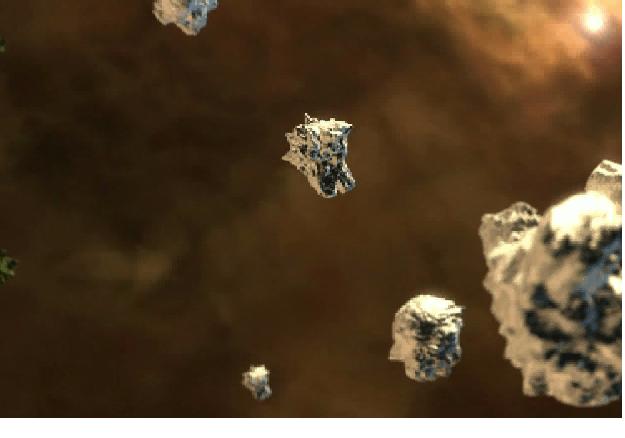
The central protostar grows, not steadily but in violent bursts and cascades (Green et al. 2006); Green et al. 2011b; Lee et al. (2011), Green et al. (2013a), (2013c) , Dunham et al. 2012; fed by the material raining from the envelope onto the disk and spiralling through the disk into the star. As planets form and the disk is dissolved by radiation from the central protostar, portions of the disk are cleared out, leaving gaps and holes (Forrest et al. 2004), (Calvet et al. 2005), (D'Alessio et al. 2005).
The Formation of a Planet
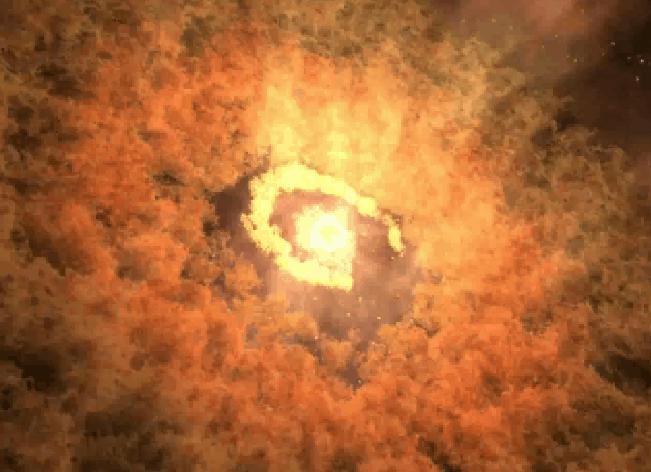
The disk which initially may have been flared like the bell of a trumpet, settles slowly onto a flat midplane and aggolomerates into bigger particles (Furlan et al. 2006). Finally the envelope and disk have almost dissipated and most of the planets have formed, although this stage may take tens of millions of years (Hartmann et al. 2005), (Uchida et al. 2004), (Furlan et al. 2007). If the central star has not built up enough mass, it will eventually stop shrinking and radiate away its remnant heat, becoming a brown dwarf (Furlan et al. 2005a). If it grows large enough, it will shrink until the core gets so hot that fusion of hydrogen into helium becomes commonplace, igniting a nuclear furnace that heats the star and its planetary system for millions or billions of years. At this stage the star is on the "main sequence," like our sun. A remnant debris disk of dust may remain or even coalesce planets while the star is on the main sequence (Jura et al. 2004).
After the star has burned most of the hydrogen in its core, it shrinks further, becoming denser and hotter. If it is massive enough, it will burn the helium as fuel. The outer portions of the star will start to puff out, perhaps enveloping some of the inner planets; in this stage the star is known as a red giant (Jura et al. 2006). When the helium has run out, the core of the star will contract even further, attempting to burn the new biproducts.
Eventually, one of two things will happen. In the case of a lower mass star (like our sun) the core will become so dense that it stops shrinking. If the star is heavy enough, the core will continue to shrink and burn heavier and denser elements until the entire core has been burned into iron. In either case, the outer portion of the star will be blown off, perhaps forming a planetary nebula as it drifts from the central core. The central core will shrink, and this time there is no fuel burning to prop it up and halt the collapse. If the star has a relatively light core, it will eventually stop contracting because of electron degeneracy pressure, forming a white dwarf star. If it is a heavier core, this will not stop the contraction, and it requires a stronger pressure at an even larger density: neutron degeneracy pressure. At this point, the core is made up almost entirely of neutrons pressed against each other. The resulting explosion of energy is so violent that it is released very rapidly; this is known as a supernova. Many of the heavier elements that were built up in the core are blown out during this explosion, seeding the interstellar medium.
If even neutron degeneracy pressure fails to hold the core together, as is the case in the heaviest stars, the core completely collapses and becomes a black hole, an infinitely dense ball of matter from which even light cannot escape.
Thinking back to the original cloud: where did the initial compression come from? One possibility is that the shock from a dying star's supernova may cause a ripple to propagate through space, and when it compresses the cloud, star formation begins once again.
Triggered Star Formation?
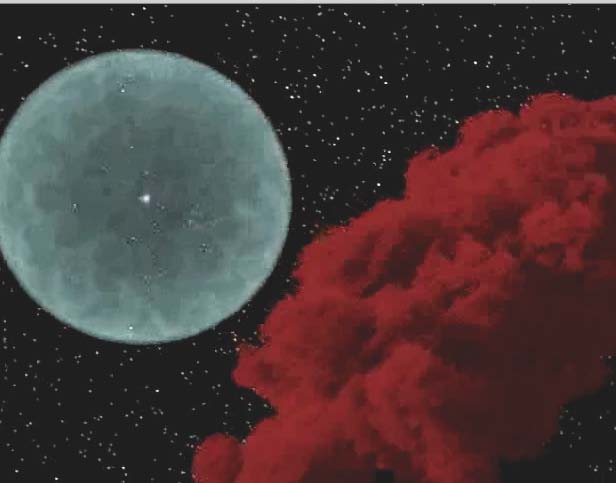
But another possibility is that high-mass young stars may stir up the molecular cloud around them. Young stars that are accreting large amounts of material fire off bipolar outflows that travel through the molecular cloud. The outflow may ionize the molecular hydrogen as the shock passes by (My Thesis, Green 2009; Green et al. 2011a; Green et al. 2013d, in prep.(Maret et al. 2009).
Popular Science Articles
Learning How to Build a Solar System: Mercury, Summer 2010 issue
Proceedings Editor
Frank N. Bash Symposium, University of Texas at Austin, October 2011
Frank N. Bash Symposium, University of Texas at Austin, October 2009
Primary Research Papers (see CV for most updated list)
An Analysis of the Environments of FU Orionis Objects with Herschel
Embedded Protostars in the Dust, Ice, and Gas in Time (DIGIT) Herschel Key Program: Continuum SEDs, and an Inventory of Characteristic Far-Infared Lines from PACS Spectroscopy
Variability at the Edge: Optical Near/IR Rapid-cadence Monitoring of Newly Outbursting FU Orionis Object HBC 722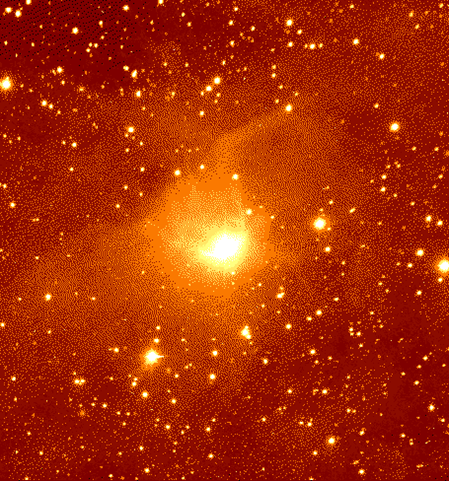
Transitional disks and their origins: an infrared spectroscopic survey of Orion A 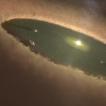
DIGIT survey of far-infrared lines from protoplanetary disks II: CO 
DIGIT survey of far-infrared lines from protoplanetary disks I 
Revealing The Millimeter Environment of the New FU Orionis Candidate HBC722 with the Submillimeter Array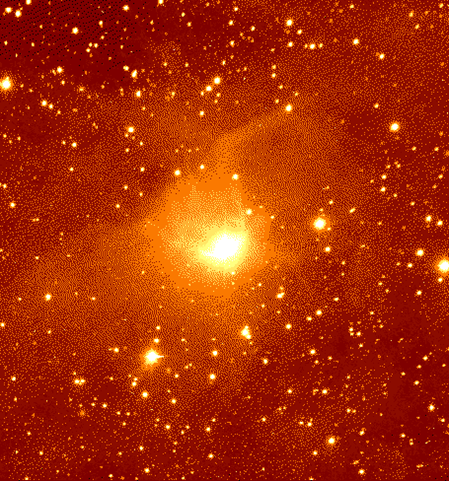
Disentangling the Environment of the FU Orionis Candidate HBC 722 with Herschel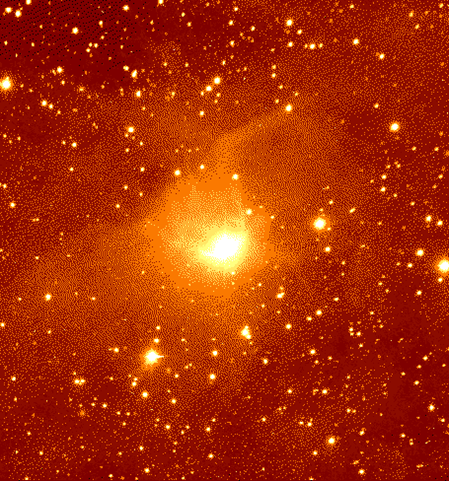
Spitzer-IRS Observations of the Varied Shocks of Cep A West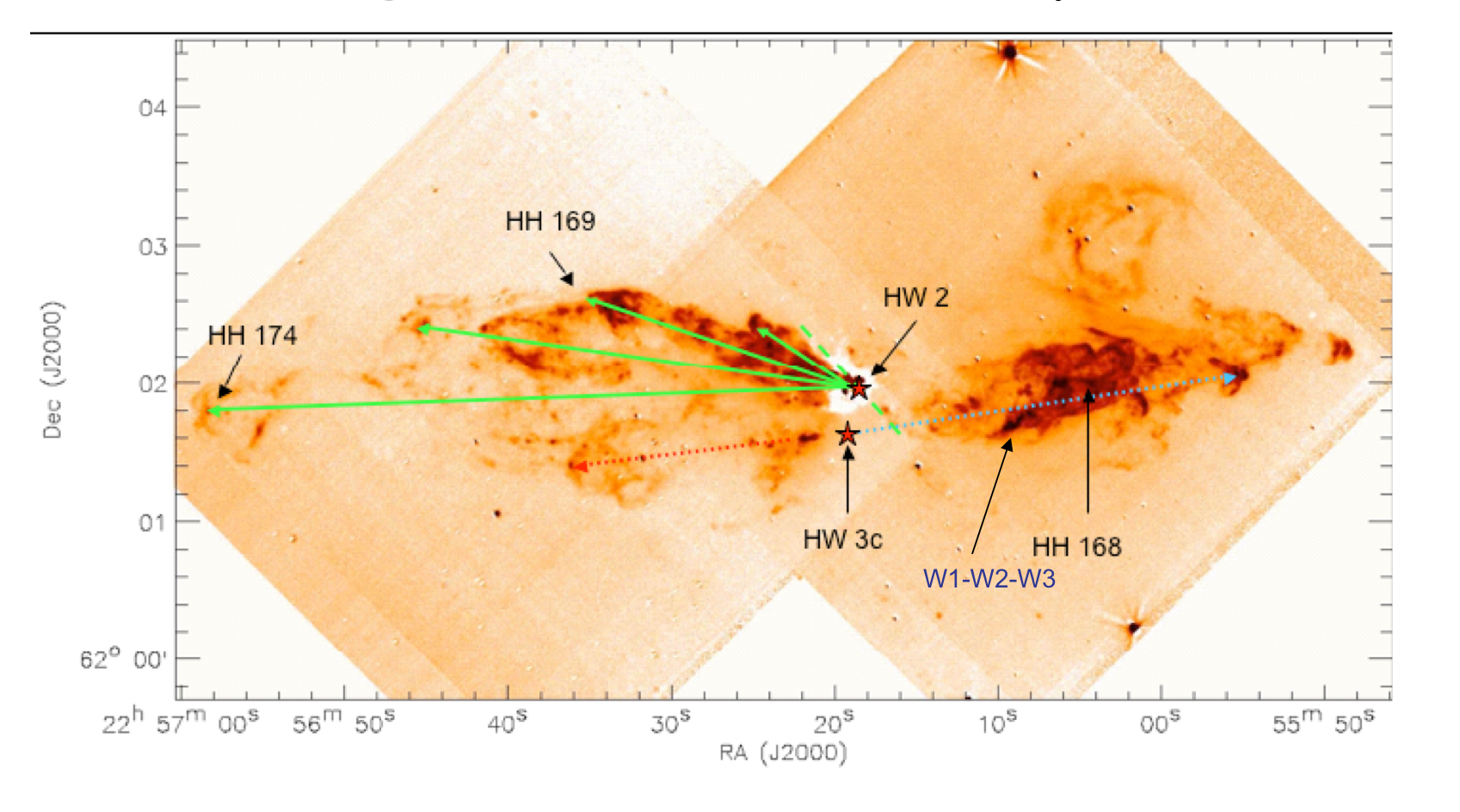
GGD37: an Extreme Protostellar Outflow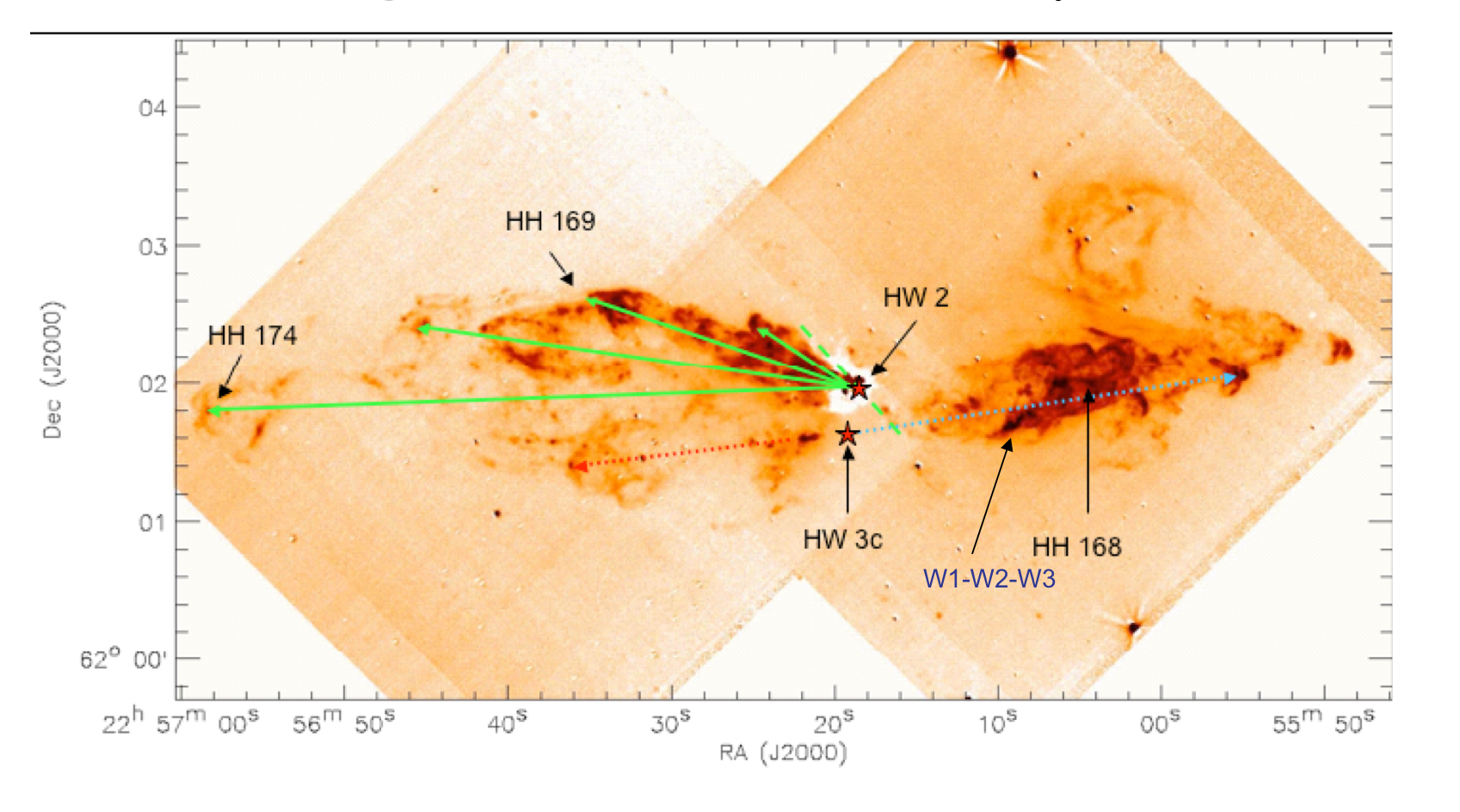
High Resolution Optical Spectra of HBC 722 After Outburst 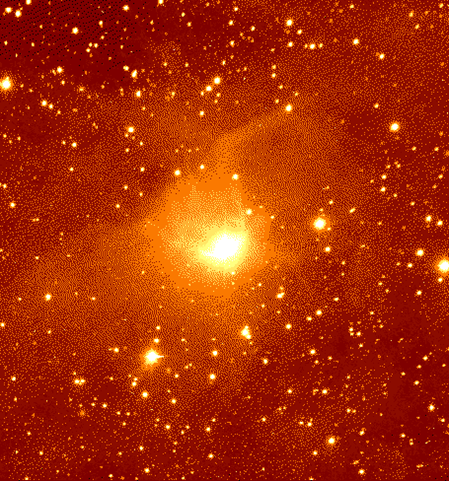
Dust, Ice and Gas in Time (DIGIT) Herschel program first results: A full PACS-SED scan of the gas line emission in protostar DK Cha 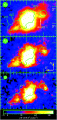
First results of the Herschel Key Program 'Dust, Ice and Gas in Time': Dust and Gas Spectroscopy of HD 100546 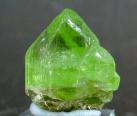
Spitzer Mapping of Molecular Hydrogen Pure Rotational Lines in NGC 1333: a Detailed Study of Feedback in Star Formation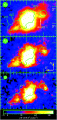
Crystalline Silicates and Dust Processing in the Protoplanetary Disks of the Taurus Young Cluster<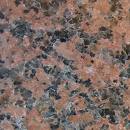
Ph D Thesis: Observations of Feedback Between Protostars and Their Natal Clouds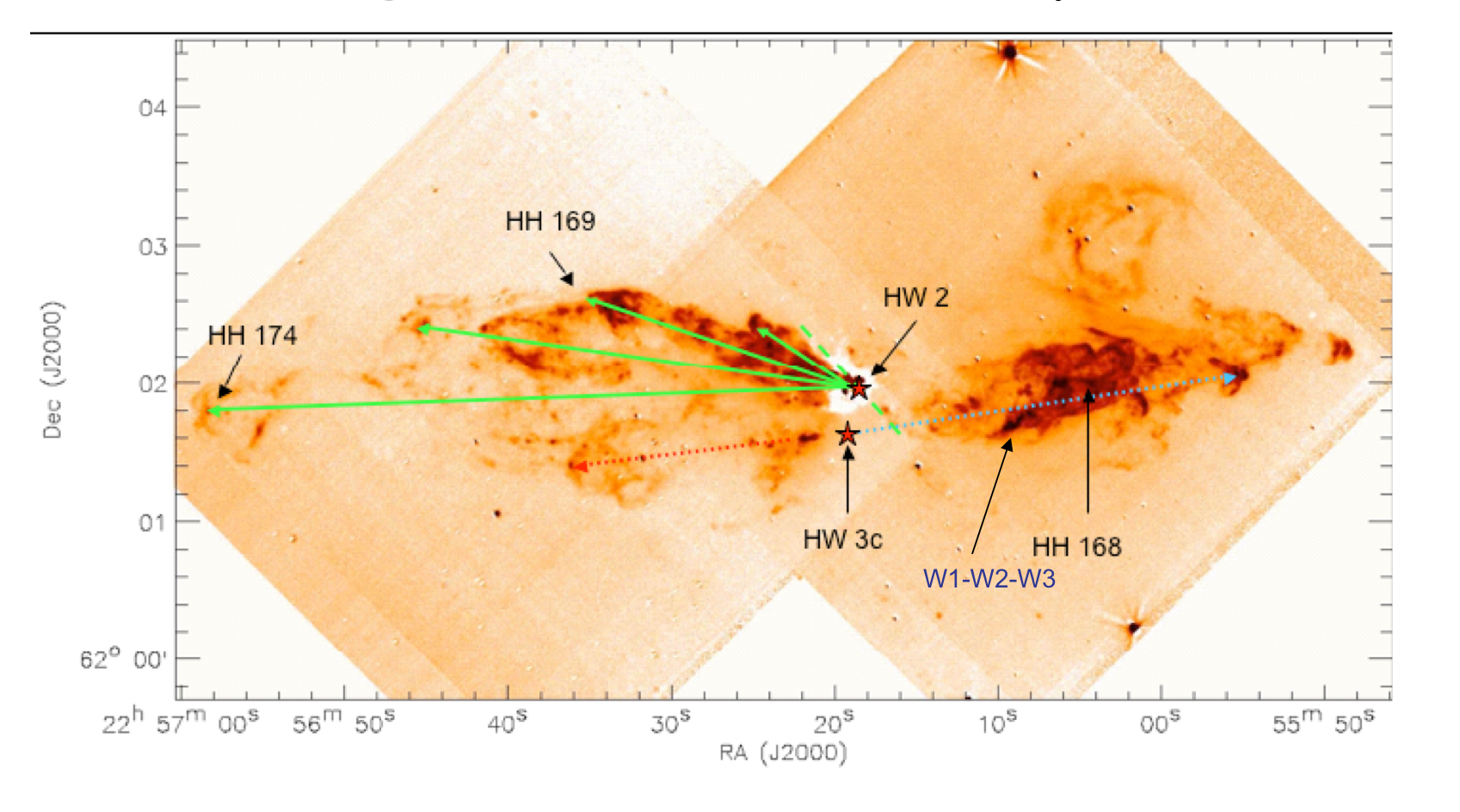
Spitzer-IRS Spectra of FU Orionis Objects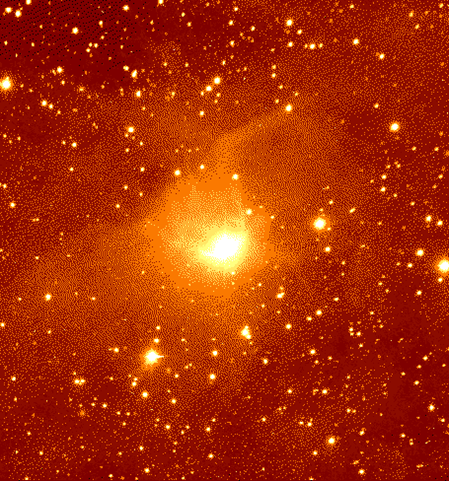
Silica in Protoplanetary Disks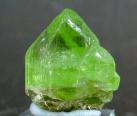
Spitzer IRS Spectra and Envelope Models of Class I Protostars in Taurus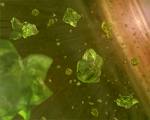
Spitzer IRS Observations of Class I/II Objects in Taurus: Composition, Temperature and Thermal History of the Circumstellar Ices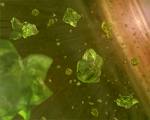
Dust Processing and Grain Growth in Protoplanetary Disks in the Taurus-Auriga Star-Forming Region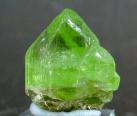
Spitzer IRS mapping of the central kpc of Centaurus A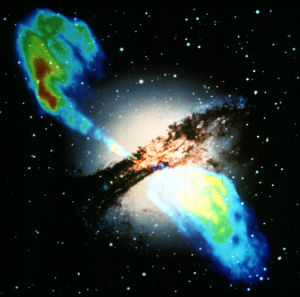
The development of a protoplanetary disk from its natal envelope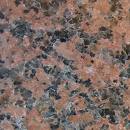
Crystalline silicates and dust processing in the protoplanetary disks of the Taurus young cluster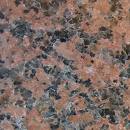
HD 98800: A 10-Myr-Old Transition Disk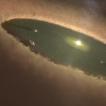
A Survey and Analysis of Spitzer Infrared Spectrograph Spectra of T Tauri Stars in Taurus
Spitzer observations of hydrogen deuteride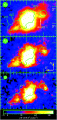
Dust Processing in Disks Around T Tauri Stars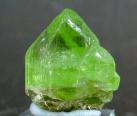
Spitzer observations of HH54 and HH7-11: mapping the molecular hydrogen ortho-para ratio in shocked molecular gas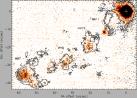
Polycyclic Aromatic Hydrocarbons Orbiting HD 233517, An Evolved Oxygen-Rich Red Giant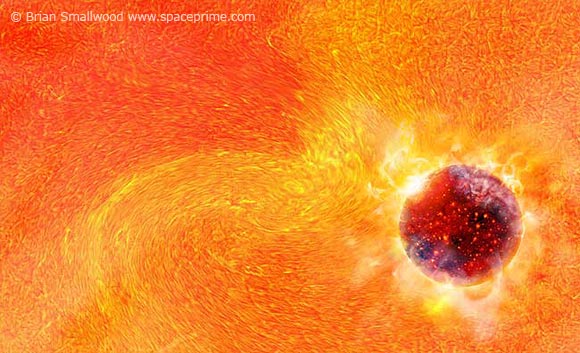
Colors of Classical T Tauri Stars in Taurus Derived from Spitzer IRS Spectra: Indication of Dust Settling
Spitzer IRS Spectra of Young Stars Near the Hydrogen-Burning Mass Limit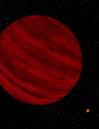
Spitzer detections of new dust components in the Red Rectangle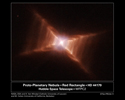
Disks in Transition in the Taurus Population: Spitzer IRS Spectra of GM Aurigae and DM Tauri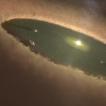
The Accretion Disk of the Lithium-Depleted Young Binary St 34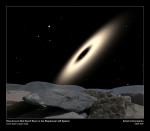
The Truncated Disk of CoKuTau/4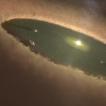
Mid-Infrared Spectra of Polycyclic Aromatic Hydrocarbon Emission in Herbig Ae/Be Stars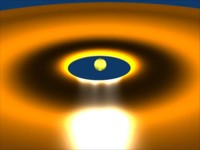
Mid-IR Spectra of Class I Protostars in Taurus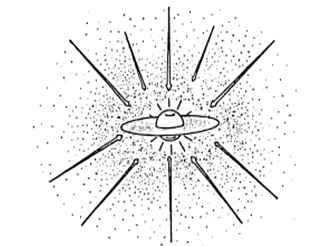
Mid-IR Spectroscopy of Disks Around Classical T-Tauri Stars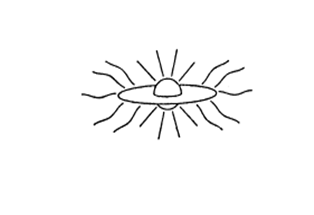
Mid-IR Spectra of Dust Debris Around Main-Sequence Stars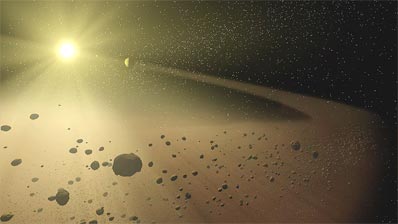
Circumstellar Disks in the TW Hydrae Association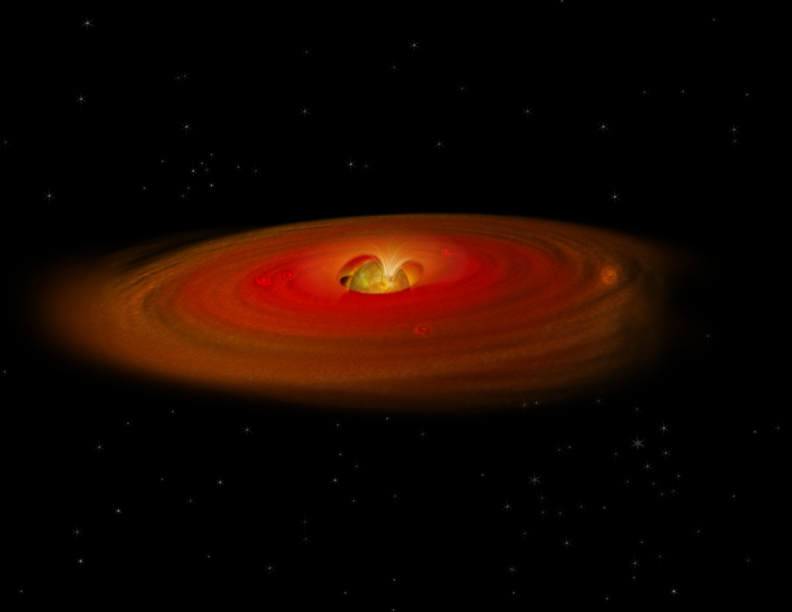
The SMART data analysis package for the Infrared Spectrograph on the Spitzer Space Telescope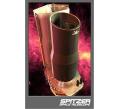
Back to the Index
This page last updated 4 June 2013 by JDG | ©2006-2014, Joel David Green. Animations provided courtesy of Robert Hurt and Tim Pyle, SSC.